The first pull on a cigarette should send you into convulsions. The brain proteins that nicotine affects are nearly identical to a receptor protein on muscle cells that tells them to contract, but nicotine doesn't affect your muscles. "Muscle proteins couldn't be very sensitive to nicotine," says chemist Dennis Dougherty. "Because if they were, smoking would be intolerable—every puff would activate every muscle in your body."
So Dougherty and biologist Henry Lester set out to discover why nicotine prefers brains over brawn. Their work may help explain why smoking is addictive, and it could enable the design of drugs to help you quit. Surprisingly, it might also lead to treatments for neurological diseases, including Parkinson's and schizophrenia. (There is no medical justification for smoking, but people who have smoked for 30 or more years are almost 50 percent less likely to develop Parkinson's disease than nonsmokers, and about 90 percent of schizophrenics smoke compared to 20 percent of the general population. It may be that nicotine helps counteract the attention and memory losses of schizophrenia.)
Nicotine hijacks a family of proteins that bind to acetylcholine, a neurotransmitter-of-all-trades. In the brain, acetylcholine is involved in learning and memory, in maintaining alertness, and in the sensation of pleasure. In the rest of your body, it's the intermediary between your nerve cells and your muscle cells, carrying commands across the synapses that separate them and setting your body in motion. So when you flex your pecs in the mirror and think to yourself, "Dang, I look good," that's acetylcholine at work.
The receptors loosely resemble molars, with five roots and a crown, and sit embedded in a cell wall like teeth in a jawbone. Each tooth has a cavity on one side of the crown—the binding site, into which the acetylcholine molecule fits perfectly. The act of binding opens a pore that runs down the center of the tooth like a root canal, allowing ions to flow and create an electrical current.
There are more than 20 known versions of the receptor. Each version is assembled from an assortment of five subunits, with each subunit running from a root up to its corresponding cusp, surrounding the root-canal pore. "The different receptors are siblings—more closely related than cousins—but not identical twins," Dougherty says. But while binding acetylcholine brings them together as a family, their different collections of subunits allow at least some of them to choose to bind various other molecules as well. This would not seem too surprising, except that other scientists had found that the actual binding site is identical: the same five amino acids arranged like the bottom and sides of a lidless box. "So this raises a fascinating question," says Dougherty. "We have two dozen different acetylcholine receptors with noticeably different pharmacologies. What's happening?"
Answering that question is a detective story 20 years in the making, complete with red herrings, cold trails, and undercover informants. There's even an unlikely hero—a frog whose unfertilized eggs can be persuaded to sprout a crop of receptor proteins on their surfaces.
It turns out that a single amino acid that's not even a part of the binding box holds the key. There's a critical spot, four amino acids away from the binding site, where changing one amino acid will allow an acetylcholine receptor to broaden its repertoire. Amino acids come in assorted sizes, and it appears that putting a bigger one at this particular spot nudges the binding box just enough to change its shape slightly. This altered shape, in turn, can accommodate other molecules—with who knows what other effects.
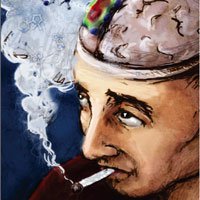
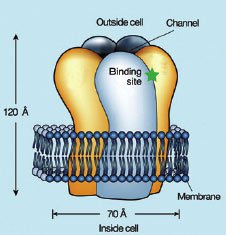
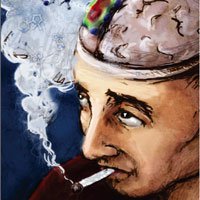
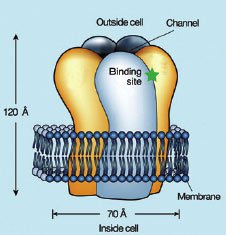