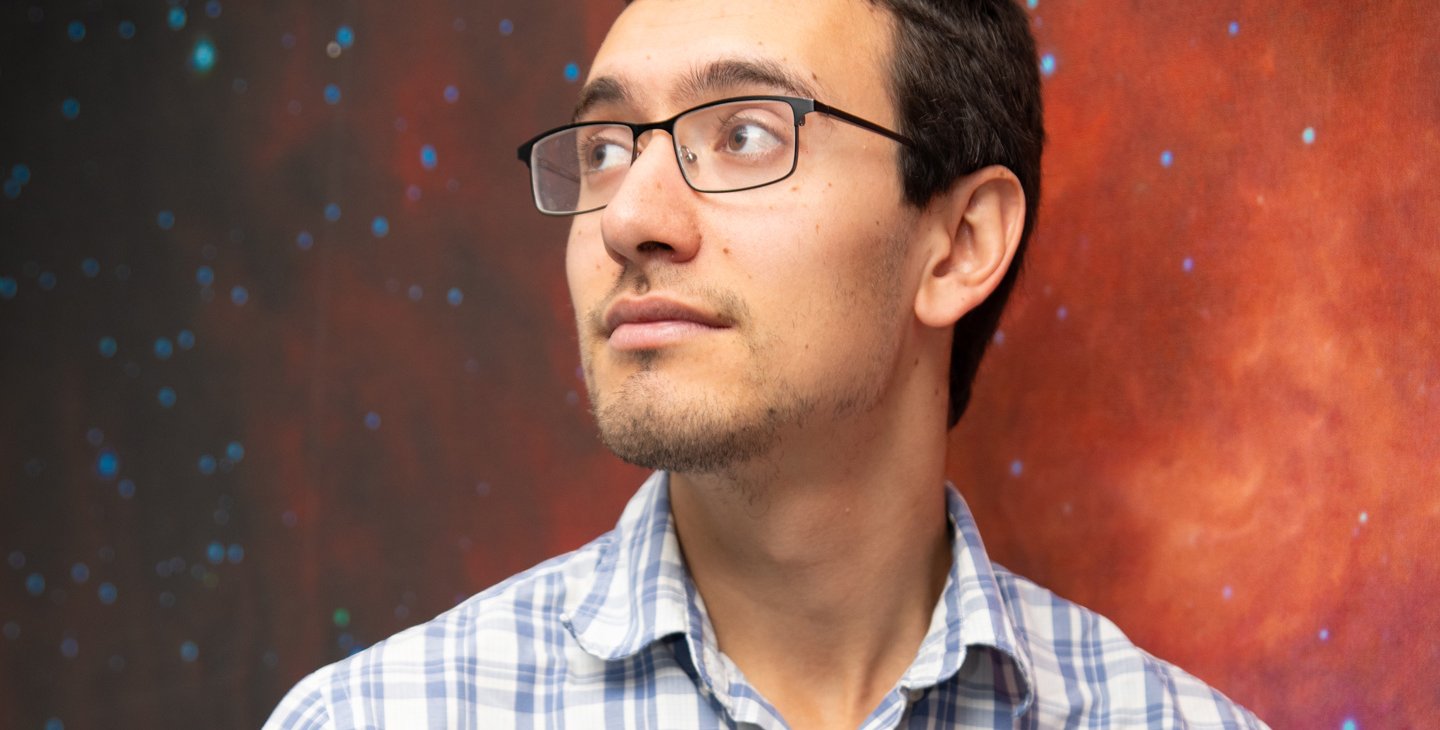
A Black Hole Hunter Arrives at Caltech
Before joining the faculty this academic year as assistant professor of astronomy, Kareem El-Badry made headlines for debunking other researchers' claims to have discovered black holes. A number of celestial phenomena that suggest the presence of a black hole—which is, as its name suggests, not visible—can be explained in other ways. For El-Badry, debunking black hole claims is all part of a quest to find actual black holes, to understand their dynamics, and to estimate their population in the universe.
El-Badry attended Yale University as an undergraduate and earned his PhD in astrophysics from the UC Berkeley. Before coming to Caltech, El-Badry held a postdoctoral position at the Harvard & Smithsonian Center for Astrophysics. He continues to hold a visitor appointment at the Max Planck Institute for Astronomy in Heidelberg.
We recently had a conversation with El-Badry to understand more about his fascination with stars and the voids of dark energy they sometimes leave behind.
What attracted you to astrophysics?
When I started college, I thought I'd be in the humanities. I came in as a philosophy major. But then, in my first year, I took an introduction to astronomy class, and that was my gateway drug. I took a lot of physics classes after that and then did summer research, first at Yale, where I was an undergraduate, and then at Caltech as a SURF student in Phil Hopkins's lab [Hopkins is Ira S. Bowen Professor of Theoretical Astrophysics]. I realized pretty quickly that I liked science more than philosophy. And I felt like there were more accessible questions in astronomy than in other areas of physics. I could pick a new research area, and within six months or a year I could be learning something new that people hadn't known before.
What makes you the destroyer of black holes?
This was never my goal. Truly. I spent much of my research time in graduate school looking for black holes of a specific flavor: those formed in the deaths of massive stars. These "stellar-mass" black holes are distinct from "supermassive" black holes found at the centers of galaxies. At the time, the only stellar-mass black holes we were aware of were in X-ray binaries. These systems include a star that is orbiting tightly around a black hole and gradually being eaten by it. As material from the star falls into the black hole, it releases a lot of energy in the form of X-rays, which we can detect because they are very bright.
I was searching for something different—non-accreting black hole binaries, which consist of a black hole that is paired with a star but with the two objects far enough apart that the star is not being eaten by the black hole. Especially since gravitational-wave detections began in 2015, we have suspected that the kind of black holes we see in X-ray binaries are not necessarily representative of the whole black hole population in terms of their masses, how they formed, their spins, and so on.
I spent several years looking for non-accreting black holes, and I didn't find anything. But other groups started publishing discoveries, so I began looking into these to improve my own search methods. And for four years or so, every single one I looked into, it turned out it was not actually a black hole. I would reanalyze their data, or sometimes get my own data. You see, it's a process of elimination to decide if you've found a black hole; you have to rule out all the other possibilities. And I found that whether or not you find the companion around which a star is orbiting has to do with how thoroughly you look. In a lot of cases, at first it looks like there's no visible companion to the orbiting star, but if you look a little closer, you find that there is one.
I think with all the objects I've written about, by now even the authors of the original discovery papers have since acknowledged that these things were probably not black holes.
Were you eventually able to find binary star systems with a black hole and a star in which the star wasn't falling into the black hole?
Yes. In the last year or so we've found two black holes that are closer to us than any others we know of. They're both separated from their star by more than the distance between the Earth and the Sun. That's much too far apart for there to be any transfer of star matter onto the black hole.
We suspect that here on Earth we are not at a particularly unusual place in the universe, so the black holes that are closest to us are probably the most common type of black holes. Some of the main things I'm working on now are finding more of them, measuring their physical parameters, observing them at all wavelengths, and trying to build evolutionary models for how they form.
How did you detect them?
Most stars are just moving in straight lines through the galaxy. But if they're orbiting a black hole or another star, they'll have an orbital motion in addition to that velocity, an extra wobble. The Gaia mission, which is being run by the European Space Agency, is measuring that wobble for something like a billion stars. They had their first data release with information about binaries about a year and a half ago, which is how we found these two black holes closest to us. That first data release was only about 1 percent of the total volume of data expected from this mission. The next big data release is a year or two away, so we feel like we'll soon know a lot more than we do now.
Just how close are these black holes to us?
The two we've found are 20 times closer to us than the black hole at the center of the Milky Way. They're in our galaxy. One is about 1,500 light years away and the other is something like 3,500 light-years from us. In comparison, the center of the galaxy is 25,000 light-years away.
The Milky Way has one big black hole at its center that is a few million solar masses in size. We think that there are somewhere between 10 million and 100 million smaller black holes that are mixed in with the stars in the galaxy. They're the corpses of stars that have already died. In terms of total mass, these small black holes outweigh the single black hole at the galaxy's center by a factor of 100 to 1,000.
Models suggest that the closest black hole to us should be only 30 to 50 light-years away. In fact, there should be a million black holes that are closer to us than the closest one we've found so far! It's just that most of them are probably not being orbited by a star, so they're difficult to find.
Where do you get your data?
I've used the Keck telescope in Hawaii and smaller telescopes in California, Chile, and Arizona—basically anything I can get my hands on. For these kinds of discoveries, we need a lot of measurements. But since we're looking at relatively bright stars in these binary systems, we don't necessarily need a big telescope. We just have to look at the same star many nights in a row. We often combine data from different observatories.
If you view the spectrum of a star at different stages in its orbit, you can see how that spectrum changes. If its companion really is a black hole, if it's not producing any light, then you should see Doppler shifting in the star's spectrum [a change in the frequency of light that tells us if an object is moving toward us or away from us], but the shape of the features in the spectrum shouldn't change at all. If there's another star there, then the spectrum will show some other changes.
How do you like Caltech so far?
For the area I work in—stars, binaries, compact objects—there are quite a few people here who are either developing models to compare to the data that I'm getting or generating the data in the first place. There are lots of telescope resources, and Pasadena is nice. So far, everything is good. I've particularly liked getting set up with my research group. Even though it's only been six or seven months, I already see the students making a lot of progress. It's nice to have that little community.
Are there other topics your group is researching?
Yes. Most projects we're working on involve some kind of binary star. There's a class of supernova that are used a lot in cosmology because they're basically all the same brightness: type 1A supernovae. We're pretty sure that unlike normal supernovae, which are caused by the collapse of a massive star, type 1A supernovae result from the explosion of a white dwarf, a low-mass star. We still don't know exactly what causes them to explode. We think the white dwarf has to be in a binary star system and the other star has to be transferring mass onto the white dwarf. But just what causes the detonation is still unclear.
My group is interested in a model for type Ia supernovae that begins when you have two white dwarfs in a binary system spiraling into one another. White dwarf stars are very dense, so they can move into really tight, fast orbits. When they get to the point where they're about to merge, something detonates one of the white dwarfs. The runaway fusion from the center of that star blows it up, almost vaporizing it, and the other star is flung out into space at its orbital velocity.
So, if you find stars that are moving in a big hurry, maybe they are former participants in a binary star dance?
Exactly. And we've now found a few of these runaway white dwarfs. They are moving much faster than the escape velocity of the Milky Way, so we know they must have formed recently, otherwise they wouldn't be here anymore.
When you trace their trajectory backwards, in some cases it leads right to the remnant of a type 1A supernova, so we think we have pretty strong evidence that at least some type 1A supernovae come from double white dwarf mergers.
When we look at the spectra of the runaway white dwarf stars, we see elements that are not normally there, which we think are the products of the nuclear burning of the companion that exploded.
If we can measure the lifetimes of these objects, we can estimate a birth rate for them and discover how often these stars are ejected from a binary system. We can compare that rate to the known rate of type 1A supernovae and see if this is where these supernovae typically come from or if there is some other mechanism.
Has your time in astrophysics changed the way you look at the night sky? If you think back to how it looked to you before you became an astronomer, is it different now?
I think it has changed some. Honestly, though, I don't look at the night sky that often. Recently I've gotten interested, just as an amateur, in some of the stars that you can see with the naked eye. Like the North Star, Polaris, is a Cepheid variable. If you measure the period on which a Cepheid is pulsating, you ought to be able to see that it grows brighter and fainter over many nights, perhaps even with the naked eye.
But if I walk you outside in the dark, can you point to the location of the two black holes you've found in the Milky Way?
I know where they are in the sky, yes. The stars they're orbiting are too faint to see with the naked eye, but they're still bright by astronomical standards. Even with a small telescope, you could see them.