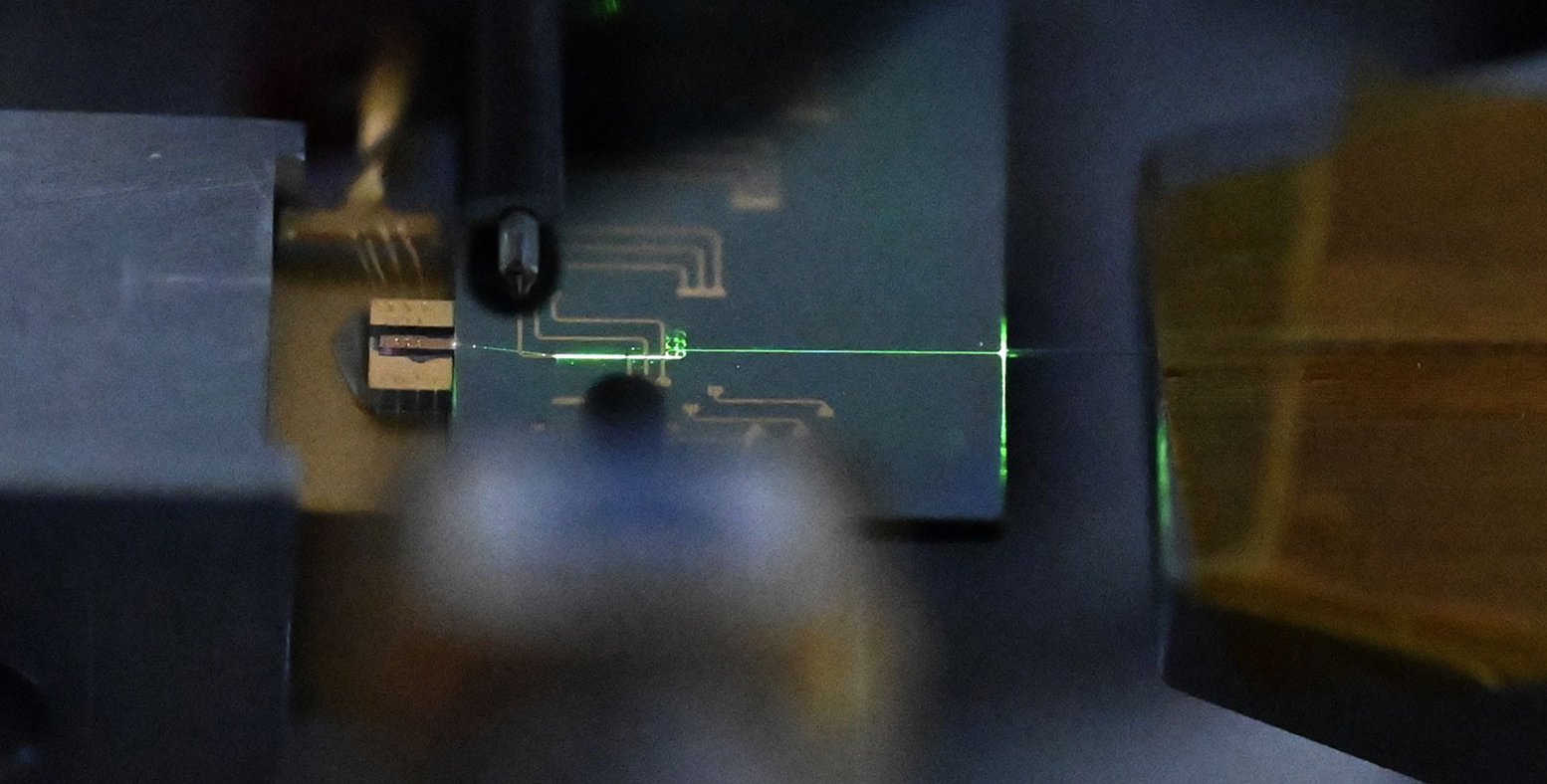
Ultrafast Lasers on Ultratiny Chips
Lasers have become relatively commonplace in everyday life, but they have many uses outside of providing light shows at raves and scanning barcodes on groceries. Lasers are also of great importance in telecommunications and computing as well as biology, chemistry, and physics research.
In those latter applications, lasers that can emit extremely short pulses—those on the order of one-trillionth of a second (one picosecond) or shorter—are especially useful. Using lasers operating on such small timescales, researchers can study physical and chemical phenomena that occur extremely quickly—for example, the making or breaking of molecular bonds in a chemical reaction or the movement of electrons within materials. These ultrashort pulses are also extensively used for imaging applications because they can have extremely large peak intensities but low average power, so they avoid heating or even burning up samples such as biological tissues.
In a paper appearing in the journal Science, Caltech's Alireza Marandi, an assistant professor of electrical engineering and applied physics, describes a new method developed by his lab for making this kind of laser, known as a mode-locked laser, on a photonic chip. The lasers are made using nanoscale components (a nanometer is one-billionth of a meter), allowing them to be integrated into light-based circuits similar to the electricity-based integrated circuits found in modern electronics.
"We're not just interested in making mode-locked lasers more compact," Marandi says. "We are excited about making a well-performing mode-locked laser on a nanophotonic chip and combining it with other components. That's when we can build a complete ultrafast photonic system in an integrated circuit. This will bring the wealth of ultrafast science and technology, currently belonging to meter-scale experiments, to millimeter-scale chips."
Ultrafast lasers of this sort are so important to research, that this year's Nobel Prize in Physics was awarded to a trio of scientists for the development of lasers that produce attosecond pulses (one attosecond is one-quintillionth of a second). Such lasers, however, are currently extremely expensive and bulky, says Marandi—who notes that his research is exploring methods to achieve such timescales on chips that can be orders of magnitude cheaper and smaller, with the aim of developing affordable and deployable ultrafast photonic technologies.
"These attosecond experiments are done almost exclusively with ultrafast mode-locked lasers," he says. "And some of them can cost as much as $10 million, with a good chunk of that cost being the mode-locked laser. We are really excited to think about how we can replicate those experiments and functionalities in nanophotonics."
At the heart of the nanophotonic mode-locked laser developed by Marandi's lab is lithium niobate, a synthetic salt with unique optical and electrical properties that, in this case, allows the laser pulses to be controlled and shaped through the application of an external radio-frequency electrical signal. This approach is known as active mode-locking with intracavity phase modulation.
"About 50 years ago, researchers used intracavity phase modulation in tabletop experiments to make mode-locked lasers and decided that it was not a great fit compared to other techniques," says Qiushi Guo, the first author of the paper and a former postdoctoral scholar in Marandi's lab. "But we found it to be a great fit for our integrated platform."
"Beyond its compact size, our laser also exhibits a range of intriguing properties. For example, we can precisely tune the repetition frequency of the output pulses in a wide range. We can leverage this to develop chip-scale stabilized frequency comb sources, which are vital for frequency metrology and precision sensing," adds Guo, who is now an assistant professor at the City University of New York Advanced Science Research Center.
Marandi says he aims to continue improving this technology so it can operate at even shorter timescales and higher peak powers, with a goal of 50 femtoseconds (a femtosecond is one-quadrillionth of a second), which would be a 100-fold improvement over his current device, which generates pulses 4.8 picoseconds in length.
The paper describing the research is titled "Ultrafast mode-locked laser in nanophotonic lithium niobite" and appears in the November 9 issue of Science. Co-authors are Benjamin K. Gutierrez (MS '23), graduate student in applied physics; electrical engineering graduate students Ryoto Sekine (MS '22), Robert M. Gray (MS '22), James A. Williams, Selina Zhou (BS '22), and Mingchen Liu; Luis Ledezma (PhD '23), an external affiliate in electrical engineering; Luis Costa, formerly at Caltech and now with JPL, which Caltech manages for NASA; and Arkadev Roy (MS '23, PhD '23), formerly of Caltech and now with UC Berkeley .
Funding for the research was provided by the Army Research Office, the National Science Foundation, and the Air Force Office of Scientific Research.